Before we get started, I’d like to thank Margarite, who’s sitting right over there, for helping me pull all this together. It’s not easy to get so many UNC faculty in one place at the same time. How many did you say we have here today, Margarite? Fifty-three? Cool. And I know you’re all busy, too, so thanks for showing up. Any questions before we start? Yes, Professor Rial? Coffee? In the corner right back there. Mind the cord. Others?
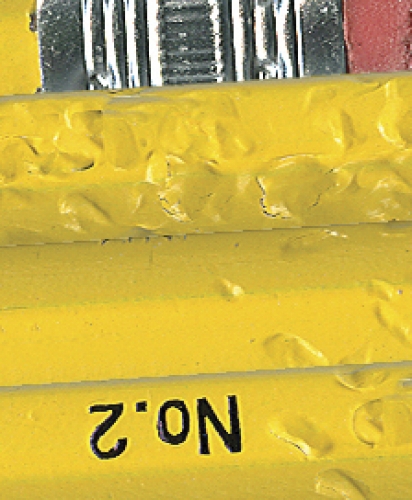
Photo by Stefan Klein; ©2008 Endeavors.
Click to read photo caption. Photo by Stefan Klein; ©2008 Endeavors.
Okay. So.
A while back, I asked all of you to help me suss out the biggest, most mysterious, and most challenging puzzles of knowledge that we face today. I wanted you to tell me about the most interesting gaps in our understanding of the world. I wanted to hear about what we don’t know. You told me, all right. You gave me a list so long that we could never talk about all of them here today. So I asked you to vote to narrow the list down to ten of the biggest, most interesting, unsolved science problems.
And today I want to tell you about those ten. Now obviously, you all know a lot more about this stuff than I do. So feel free to chime in and help me out. Everybody ready? Good. Let’s start with number ten.
10. How can we be so genetically similar to, yet so different from, chimpanzees?
This question was sent to me by Brian Hogan in the Department of Chemistry. He’s sitting right over there in the back row.
Depending on which study you read, human DNA and chimpanzee DNA seem to be somewhere between 96 percent and 98.5 percent identical. Yet you and I don’t sleep in trees, or groom each other compulsively, or — yes, Brian? You want to explain it? Okay. Go for it.
“Thanks, Jason. Here’s what I’m getting at: what is it about that 1–2 percent difference that allows humans to mathematically determine the relative position of an electron in an atom, but makes chimps sling poop at passersby?”
Um, okay. Thanks, Brian. Now, correct me if I’m wrong: you’re not angling for a discussion on evolution versus creation science, right? You just want to know how the genes work. Right.
Humans are strikingly similar to a bunch of different critters — genetically, at least. Sixty percent of human genes are fundamentally the same as fruit fly genes, and somewhere around ninety percent of our genes are the same as mouse genes. We don’t have tails, but we have the same genes that mice have for building them. (Humans almost never switch on our tail-building genes.) Mice have a certain gene they use to build eyes. Fruit flies are more than happy to use that mouse gene to build their own eyes. So our genes are flexible, and at least some of them are generalists.
But small genetic variations can make for big differences between species, and even among members of the same species. All of us humans are around 99.9 percent genetically identical — that’s according to large-scale genome sequencing projects. So, genetically, you’re only about one-tenth of one percent different from Einstein and Shakespeare. Mom would be proud.
You’ve also got something called noncoding DNA. About six feet of DNA are wrapped up in each of your cells. We used to think that only about one inch of it actually did anything; the other five feet eleven inches seemed to be junk. Now scientists are starting to think that our one inch of working DNA, along with all the proteins it produces — and RNA, and some other cellular cousins that we don’t have time to get into today — are just our nuts and bolts, and that what we once called junk DNA actually holds our blueprints and the systems that control how we’re built.
So it’s starting to look like genes are a lot more complicated than we thought. A given gene may do a whole lot more than make one protein or control one process. Scientists once speculated that we’d need at least a hundred thousand genes to build a human. But we’ve only got about twenty-two thousand. Some worms have more than that. A lungfish has forty times more DNA than you or I have. In a given gene, different combinations of coding DNA can become active at different times to produce different proteins, and that helps explain how humans can be so complex with relatively few genes. But we still have no idea how our cells “know” which parts of a gene to pay attention to at any given time, and we really don’t know what determines which genes get switched on or off at specific times and in specific places.
Researchers have identified a few genes related to brain development and speech that look like they took one evolutionary road in humans and another in chimps. It wouldn’t be ethical to remove one of those genes in a human or chimpanzee to see what happens. The best we can do is to keep working on big-picture comparisons of human and ape genes. But our next question involves some science that might one day help us figure this chimp question out.
^ back to list
9. How does development evolve?
This one was sent to me by Bob Goldstein from over in the biology department — hey there, Bob; thanks for coming — and I’ll admit that at first, I didn’t get it. But Bob is patient, and the gist is this: all organisms — from butterflies to wombats to you and I — develop, which means that we begin life as, say, a fertilized egg cell, and end up as a fully-formed insect, or an Australian marsupial, or the quarterback for the Green Bay Packers. And all organisms have also evolved over time: our forms have changed, often quite a bit, when compared to our ancestors of billions of years ago. Throw the study of development and evolution together and you get a relatively new branch of biology called evo-devo. I know it sounds like an 80s band, but bear with me. According to evo-devo, development helps steer evolution: if the history of life on earth is a long road, development has spent a lot of time in the driver’s seat.
Evo-devo research is starting to explain how evolution happens. It turns out that a complex, completely new structure — say, a butterfly’s wing — doesn’t sprout up just because a bunch of ancestral insects arbitrarily happened to have just the right genetic mutations at just the right time and place. In other words, it didn’t take new genes, or radical new developmental plans, to make wings where there were no wings before. Instead, those ancestral insects only had to slightly modify their developmental plans and tweak a few genes that they already had. Wings didn’t appear overnight, of course. But evo-devo is starting to show that new structures such as wings may have arisen from an organism’s existing genetic potential.
Another big point: we don’t need a whole gaggle of genes to make new stuff. For example, a single gene called BMP4 helps determine which parts of an embryo will become the back and belly. But BMP4 also determines whether a finch has a broad beak or a long one. It also specifies whether a cichlid fish is going to have a short, thick jaw for crushing mollusks, or a longer one for sucking algae. One gene, switched on at different times and places, may build flippers in whales and arms in football players.
Evo-devo is changing some thinking about major evolutionary steps, too. Take the transition from life in water to life on land. It didn’t necessarily happen because fish suddenly grew hands and feet. It turns out that fish had the potential to grow those appendages long before any fish dragged itself onto dry land. Evo-devo suggests that it took both the right environment and key mutations to a few existing genes to make fish take that one giant step.
So, Bob, have I done your question justice? I know evo-devo is still a fairly new field. Anything you want to add?

“Well, very few living biologists have been trained thoroughly in both of these fields. Some come from the evolution side: people who actually get to do some of their science in beautiful outdoor locations. Others come from the development side: nerds like me who stay in the lab working mostly at the minute level with molecules. So that’s a challenge. But it’s starting to change as interest in evo-devo grows.
“We don’t have a lot of general rules of evo-devo yet, but we have the tools to make the important discoveries. And the answers will fill a big gap in our understanding of how the incredible diversity of organisms we find outside came about.”
Thanks, Bob.
What’s that, Dr. Rial? We’re out of coffee? Okay. We’re on it. Let’s move on to number eight.
^ back to list
8. What caused so many abrupt changes in the climate of the past? Are we experiencing something similar now?
Well, this is technically two questions, but you all voted for it, so I guess that’s okay. Jose Rial sent this to me. He’s in the geology department, and one of the things he studies is climate change as it relates to the history of the earth. Oh, and he’s waiting for coffee.
Let me see if I can stumble through this one: up until about twenty years ago, we were sure that Earth’s climate changed gradually. It got pushed slowly one way — by, say, regular, predictable changes in Earth’s orbit — and then gradually pulled back the other way, in a nice, neat cycle. Sure, it might have to account for some unpredictable hiccups along the way, but the point is that it was supposed to react very slowly.
But then paleoclimatologists started finding climate patterns, both in the ancient and the more recent past, that didn’t jibe with the conventional wisdom. They found a bunch of abrupt climate swings — big changes in average temperature, or puzzling patterns of storms, floods, and droughts, over a large part or parts of the world — that happened fast: sometimes within a decade. So fast that the earth, and most things on it, had trouble adapting.
We don’t know exactly why these abrupt changes happened, but we have a few suspects. The oceans store a lot of heat and move it around. Then there’s the atmosphere and its soup of temperature, humidity, cloudiness, and wind. Ice and snow reflect sunlight and heat, and sea ice keeps the oceans from giving heat back to the atmosphere. The output of the sun fluctuates. Any and all of these are fair game.
In 2002 the National Academy of Sciences issued a book on abrupt climate change. They subtitled it Inevitable Surprises. They theorized that abrupt climate changes happen when the earth’s climate system gets pushed across a threshold, either by a sudden trigger — say, a massive volcanic eruption — or by combinations of more gradual forces. It all works, they said, like a canoe: you can lean out over the side a bit, but keep leaning further and eventually you’re going to turn the boat over.
Professor Rial tells me there’s something called the Younger Dryas cold interval. Had you been living in the Northern Hemisphere around eleven thousand years ago, you would have been fairly happy to find that things were warming up. The earth was thawing out from an ice age. But for some reason, things got cold again, fast. Within about twenty years, the average temperature in the arctic regions had dropped by about twenty-five degrees Fahrenheit. It was almost cold enough to kick-start the glaciers again. This cold interval — which climatologists sometimes call the Big Freeze — lasted about a thousand years, and then it was gone as fast as it had come. But it may have sealed the doom of some of North America’s biggest land mammals. Saber-toothed cats, mammoths, and mastodons all went extinct around the end of the Big Freeze.
A drought that came out of nowhere and lasted for decades may have been partly responsible for the end of the classic Mayan civilization in the ninth century. And your grandparents may have lived through an abrupt climate change: the Depression-era Dust Bowl drought in the United States.
Now, I’m not trying to be sensational, but any of this stuff could happen again. And some scientists believe we may be experiencing another abrupt climate change right now. This time, some of them are blaming global warming. That’s right: something we’ve all begun to think of as a climate disaster may actually cause another, separate climate disaster. Not all climatologists think that global warming will trigger an abrupt period of flooding, or drought, or even another ice age, but the record shows that past abrupt climate changes have tended to happen when some force was altering the climate itself — just like we seem to be doing right now.
Professor Rial has done some research of his own on this stuff. He proposed a mathematical model to account for a particular kind of abrupt climate change that shows up repeatedly throughout Earth’s climate history: sudden and fast warming followed by much slower cooling. I’m not going to try to explain his model here today, mostly because I don’t understand a word of it. But I will ask Professor Rial to stand up and give us the bottom line. Would you mind, Dr. Rial? Thanks.
“Okay. Well, since many of these abrupt warming events have happened often in the recent past, it is reasonable to expect them in the near future. But what’s different right now are the unprecedented concentrations of CO2 and methane we have managed to produce, and the unprecedented rates at which we’re still emitting these gases. Nothing like this has happened at least during the last million years. As paleoclimatologist Wally Broecker would say, we are poking a sleeping dragon in the eye. Get ready for the blast!”
Thanks again, Professor Rial. Next question:
^ back to list
7. Are there too many humans for one Earth?
I got this question, stated a slightly different way each time, from four different people, in biostatistics, biology, environmental sciences, and religious studies. It seems that many of you worry that we may run out of some of the things we rely on to live.
Is Jonathan Boyarin here today? Oh, hi. Good to finally meet you face to face. Dr. Boyarin is a cultural anthropologist from the Department of Religious Studies. He’s convinced that we’ve reached or maybe already surpassed Earth’s capacity to sustain the number of humans we now have at our current levels of resource use. He told me that we have no idea how we could scale our consumption back with the least possible amounts of suffering and injustice. He insists that just thinking about local sustainability won’t do the trick: we need a game plan for global sustainability, but we don’t know how to best organize that.
Dr. Boyarin, I think I’m in over my head here. These questions are almost beyond the scope of what we can talk about today. Can you help me out?
“Well, I’ll try. You’re right in that nobody can take on this whole question, and it’s not just a question for academic specialists. But we can’t shrink from it, because there is a real world outside us that puts real constraints on us.”
Okay. So where do we start?
“Once upon a time we believed that the major differences between human groups were national differences — differences of language and other aspects of culture — and that these differences could be managed by giving each nation sovereignty within its own territorial state. But now it seems that that organizational scheme is inadequate when it comes to many of our most pressing problems. Group differences aren’t neatly contained within states, and human problems need to be dealt with at levels both larger and smaller than states.”
Can you give us an example?
“Sure. Here’s one that I’ve studied and which I care about deeply: Israel and Palestine. The population is conventionally divided into two national camps, cast in a struggle to the death over a single territory. No one reasonably expects those two strong collective identities to disappear or even weaken any time soon — nor would that necessarily be a good thing. But all life in that region is threatened by overuse of highly limited supplies of fresh water and other basic resources. Short of dissolving all group boundaries, can we find ways to mobilize people to resolve this shared threat?
“That’s just one example. But I think that if there’s to be any future that includes us, we have to learn to communicate better now. So over the next few decades, I hope we’ll come to share a realistic vision of our common predicament without trying to dissolve our cultural differences.”
Thanks, Dr. Boyarin. Did someone have a question just a minute ago? Oh. Hi, Woody! Woody Chambless, folks, from over in Biostatistics. He’s my father-in-law. But let’s hear him out anyway. Go ahead, Woody.
“Well, the Scottish philosopher Adam Smith once wrote that the striving of all individuals for their own private good would bring about general well-being. But when I think about war, overpopulation, and pollution, Smith’s idea starts to seem pretty flawed. So I would reword your question like this: how do we balance the wants and actions of the individual versus the needs of the common so that all might survive and thrive?”
Okay, good. Thanks, Woody. This is pretty sobering stuff. We’ve got a rapidly growing world population, increasing rates of consumption, and resources that are dwindling. When the United Nations Environmental Program released a global environmental outlook last year, the bottom line was that “the bill we hand our children may prove impossible to pay.”
^ back to list
6. How did the universe come into being and assume its present form?
Laurie McNeil and Laura Mersini-Houghton from over in Physics and Astronomy sent me this question. But they framed it with two more questions: “What was there before the Big Bang? And does that question have any meaning?”
Now, if you already have trouble sleeping at night, don’t start studying theoretical cosmology. It’s full of questions that just lead to more questions. And the math is really hard, too.
The ancient Hindus believed that the universe was a cosmic egg that expanded from a concentrated form. That’s not too different from what we think we know now. The Big Bang, so the theory goes, was the explosion of a very hot, very dense, and very small body of matter that gave rise to our universe some thirteen-odd billion years ago. We’re used to thinking of the Big Bang as ancient history. But in a sense, we’re all living in the Big Bang right now: the universe is expanding as we speak. So it must have been smaller yesterday. Last week it was smaller still. Follow this logic far, far back, says classical Big Bang theory, and you’ll see that everything in the universe — including all matter, gravity, electromagnetism, and the forces that hold atoms together — must have once been squeezed into a point so infinitesimally small that it had no dimensions at all. And that little twist of logic is where most us quickly ask our server for the check.
But most of us aren’t physicists. Physicists have faith. They have to. They know some of their theories, the Big Bang among them, aren’t perfect. But the best theories in cosmology are the ones that can’t be easily disproved by what we already know — or, in some cases, by what we already assume. The Big Bang plays nice with general relativity, with the cosmological principal, and with our other observations — prerequisites for any theory of the origin of the universe. And it may be that when we learn enough about something called quantum gravity, then we can patch up any holes in our understanding of the Big Bang — and what, if anything, came before it.
That’s not to say that the Big Bang is the only plausible origin-of-the-universe theory. Professor Mersini-Houghton told me that all it would take to challenge faith in the Big Bang is for another theory to come along that explains the observed universe just as effectively. The second-place horse right now involves a cyclic universe — one that expands and contracts continually. Whatever we learn about quantum gravity may eventually make the cyclic universe theory the front-runner. But for now, most physicists feel that the Big Bang is the best horse.
Professor Mersini-Houghton, are you here today? Hi there. Am I getting any of this right?
“Hi, Jason. You’re right that the theory of Big Bang inflation agrees exquisitely with our observations. But just remember that nearly perfect agreement with the data does not by any means constitute proof.”
So physicists have faith, but they aren’t necessarily sentimental?
“Right. The best science usually comes out of scrutinizing our most cherished principles and theories.”
There you have it. Some of these cosmological questions border on the religious and the spiritual. And in a way, so does our next one.
^ back to list
5. What is the basis of consciousness?
Here’s another question that was sent to me by more than one person — Edward Perl in cell and molecular physiology, and Jesse Prinz in philosophy. That’s a good sign that it’s a humdinger.
It’s an old question to philosophers, but a newer one to scientists. Plato thought about it. In the 1600s René Descartes said that body and mind must be made of different stuff: the body exists in time and space; the mind exists only in time. But today science says no: body and mind are different aspects of the same thing. Consciousness comes from the way the brain’s neurons work and the way they’re organized.
But we have no idea how consciousness works. Historically, Science magazine has said, studying consciousness has been “a dubious career move” for anyone who didn’t already have tenure (and maybe a Nobel, to boot). But that’s starting to change.
Let me point out Dr. Perl: he’s sitting right over here at the end of the second row on the left. His background is in medicine, and he’s been studying this stuff for far longer than I’ve been alive. And over there near the back corner is Dr. Prinz. Hi, Jesse. I like the Ramones shirt. Dr. Prinz is one of a relatively new crop of philosophers who are applying hard scientific evidence to the way they think about philosophy. He’s done a good bit of thinking about consciousness, too.
Somehow, when you smell a tiger lily or look at the Mona Lisa or listen to “Sheena Is A Punk Rocker,” certain neurons and glial cells in your brain “fire” and help you recognize and make sense of what you’re smelling, seeing, or hearing. When you see something red, you somehow experience the color red, rather than some other color or no color at all. You somehow turn tiny electrochemical impulses into your experience of the world — and in a sense, into you.
We can use tools such as MRI and PET scans to pinpoint areas of the brain that are involved in sensory experiences. We see which parts of the brain light up, so to speak. But as Dr. Perl told me, we still have no idea what’s behind that process inside the brain. How could electrochemical activity produce consciousness?
Dr. Perl’s research involves an aspect of consciousness that we’d like to think we could do without — pain. All of us have neurons that are involved in sensing it. Somehow, when a toothache or a tetanus shot causes those neurons to light up, we hurt. We don’t know for sure whether our brains have neurons specifically dedicated to pain, but Dr. Perl tells me that we’re beginning to think that our brains process pain information in several regions that operate in parallel.
Lucky for us, consciousness isn’t all pain — it includes wakefulness, perception, reflection, simulation, our sense of personal identity, anything running through our thoughts at any given moment, and maybe lots more. It may be that different regions of the brain generate different aspects of consciousness. People who have injured their brains can have consciousness-related problems that seem limited to the functions controlled by the injured brain area.
Dr. Prinz described consciousness to me like this: your senses are always taking in lots of information. You only pay attention to a small subset of what you take in — your brain broadcasts that small subset to its short-term storage centers. But that’s only part of the picture — right, Dr. Prinz?
“Right. Essentially the same kind of thing happens in my laptop, but I doubt my laptop is conscious. Consciousness occurs only when attention is underwritten by specific kinds of neural processes.”
Okay. So what do we think those neurons actually do?
“Well, I think the best current account goes something like this: when we attend — that’s psychological jargon for ‘when we pay attention’ — certain kinds of neurons, called fast-spiking neurons, become more active. They send out signals that cause some of the cells in our perceptual pathways to start firing in synchrony. This synchronic firing allows those cells to send signals that can be received by the brain’s working memory — it allows one area of the brain to talk to another. And it may be that this synchrony is essential for consciousness. If it is, we’re within reach of identifying the neural correlates of consciousness.”
Sounds like that might have some pretty big philosophical implications.
“Well, maybe. Philosophers are more interested in why brain events — which are changing patterns of neurochemical activity in populations of neurons — feel like anything at all, rather than nothing. I don’t think that finding the neural correlate answers that question, but it may help us answer why we ask that question in the first place. What is it about the way that we introspect our conscious states that prevents us from discerning that they’re just brain states? Typically, people have a problem with the idea that minds are just brains.”
Okay. Good stuff. Thank you, Dr. Perl and Dr. Prinz. Next question:
^ back to list
4. Can we find a sustainable energy source?
This one comes from Tom Meyer in the Department of Chemistry.
Most likely, the electricity that runs everything in your house comes from coal that was formed three to four hundred million years ago. U.S. coal supplies may be good for another 100 to 250 years, which just might give us enough time to find a replacement.
Ideally, our new energy sources would be renewable, efficient, and would cause no long-term damage to the earth. There are a few different candidates — solar, wind, geothermal, oceanic, and maybe nuclear. (There’s some debate about whether nuclear energy is sustainable.) But right now we know two things: we don’t have a magic-bullet solution, and no matter what we do, we’re going to have some growing pains.
Solar energy may end up being a major player. Sunlight is plentiful, and humans will be long gone before the sun burns out. Solar really became viable in the 1970s, when oil and energy crises coincided with technological improvements that lowered the cost of converting sunlight to electricity. When oil prices dropped again, funding for solar research dried up, and the public largely forgot about it. Now it’s the world’s fastest-growing energy technology, and it supplies about one half of one percent of the world’s total primary energy supply. But solar energy costs more to produce than almost any other electrical source. It’s obviously only available in the daytime, so solar power has to be stored, and it tends to work best as a complement to another power system. And about 4–12 percent of solar’s energy is lost in the conversion from direct current, which solar cells produce, to alternating current, which is the way we use it.
Wind power is another fast grower. It accounts for just over 1 percent of the world’s total primary energy supply, but many European countries are now getting a good bit of their electricity from the wind: Spain and Portugal rely on it for 9 percent of their production; Denmark for 19 percent. Much of the cost of wind power is up front — those huge wind turbines aren’t cheap. But some argue that because wind power doesn’t pollute or contribute to global warming, it’s cheaper in the long run than almost any other energy source. Right now, wind can be a good complementary source to supply up to about 10 percent of total electrical demand. One study found that the wind could produce over five times more power than what we now use, worldwide, from all energy sources. Whether we adopt it on a larger scale depends on what the economy and environment will allow.
Geothermal energy taps steam, hot water, or heat from deep within the earth. It’s relatively clean, safe, and sustainable. MIT researchers calculated that with a few technological improvements, geothermal energy could some day have the potential to supply all of the world’s energy needs. But funding for research and development have been relatively low. The trick is to figure out how to get high production out of a particular site without cooling that site off too rapidly. Right now, geothermal supplies less than 1 percent of the world’s total primary energy.
The ocean’s surface waves, tides, and currents may hold some of our future power. No one has put any large-scale production into place, though Portugal, Scotland, and England have plans for wave farms, and there are a handful of tidal energy test sites around the planet. The U.S. government has issued almost fifty permits for ocean-based energy projects, mainly granting the right to study feasibility, but not to build anything. But the power-generating equipment would be expensive to build and to maintain, and there are environmental concerns, too: much of the technology is new and relatively untested, so there may be long-term consequences beyond the obvious impacts to areas that contain equipment. Researchers hope that ocean power would eventually be as cost-effective as fossil fuels. The Electric Power Research Institute thinks that, at best, oceanic energy could only supply about 6.5 percent of our current needs.
Many scientists argue that nuclear power is sustainable: we can get scads of energy out of a relatively small amount of fuel; nuclear power plants don’t produce much in the way of CO2 emissions; and the waste is minimal and well-contained. But nuclear waste doesn’t sit too well with most folks. Then there’s the potential for power plant accidents and sabotage and for an increase in nuclear weapons as more countries adopt the technology. Thorium may be a better source of nuclear energy than the uranium we use now. It produces less waste — no waste, in some reactor designs — and there’s more thorium than uranium in the earth. But as long as uranium remains relatively easy to mine, we probably won’t put much effort into developing thorium-based reactors.
Fusion, which is power generated by joining two atomic nuclei, has been a kind of pie-in-the-sky energy source for fifty years or so. (Nuclear power plants produce energy by fission, which splits atomic nuclei.) The largest planned fusion reactor, called ITER, could go online as early as 2016, in a multinational effort that will try to heat plasma to ten times the temperatures found in the core of the sun. But industry people say we’re still at least a hundred years away from having commercially viable fusion energy. Or as Dr. Rial told me, fusion has been the energy of the future for many years, and it probably will be for many more.
But there are other possibilities. Tom Meyer has done a lot of green chemistry research here at Carolina. He wants to use sunlight to force water to give up its hydrogen, which could then power a fuel cell. He feels like this will probably play a small role in our energy future.
That brings up an important point: whatever we do, it’s unlikely that we’ll be able to rely on only one or two energy sources. As far as we can tell, we’re probably going to need to hitch our energy cart to a few different horses if we want to keep living the way we do today.
How did I do, Dr. Meyer? Can you help me wrap this one up?
“Sure. Finding new energy sources and learning how to use energy sustainably without enhancing global warming will arguably be the central issue for mankind in the near-to-medium-term future. How do we learn how to use what we know about technology, economics, and public policy to reach this brave, new, sustainable world?”
Thanks, Dr. Meyer.
^ back to list
3. What is most of the universe made of?
This one was sent to me by Laurie McNeil and Reyco Henning in Physics.
The stuff we can directly observe is fairly easy: we’ve nailed down the compositions of planets, stars, faraway galaxies, you name it. But about 95 percent of the universe is made of stuff we can’t see — some kind of matter and energy that’s not playing by the rules. It’s invisible to every kind of telescope. But we know it’s there.
We know because the universe doesn’t contain enough visible mass — that is, stars and other objects — to account for the amount of gravity it seems to have. If our theory of gravity is right, then the universe contains more mass than we’re able to see. To help explain this, physicists came up with something they called dark matter.
Dark matter, as physicists now realize, can’t be made of the same atomic stuff — protons, electrons, and the like — that makes up you and me and everything we can see. Many physicists suspect dark matter will turn out to be some bizarre kind of particle. Some are even trying to create it in the lab. And they’re optimistic that we’ll soon know what it is.
Dr. Henning studies experimental particle astrophysics. He tells me that there are three possible explanations for dark matter. Dr. Henning, if I run through them one by one, can you give us the lowdown on each?
First, our understanding of gravity might be incomplete. If it is, dark matter might just be a misinterpretation. Is that a fair summary, Dr. Henning?
“Not bad. The problem with that idea is that nobody has formulated a reasonable alternative theory of gravity that explains all our experimental and observational data. And our best theory of gravity, general relativity, passes all the experimental tests we’ve thrown at it.”
Okay. Possibility number two: our universe might be relatively full of things that we just can’t see, even though they’re made of normal matter — cold gas, dead stars, and other objects that don’t emit or reflect enough light to be visible.
“That sounds like the simplest solution, but there are serious problems with it. Some of our most verified theories in cosmology — such as our theory about the origin of primordial elements — would fall apart if dark matter turned out to be normal matter.”
And now possibility number three: maybe some kinds of particles don’t interact with electromagnetic radiation. For example, some physicists hypothesize the existence of Weakly Interacting Massive Particles — or WIMPs — that may have been produced in huge amounts during the Big Bang. What about WIMPs, Dr. Henning?
“WIMPs are, arguably, the simplest explanation of dark matter. Explaining them requires the least amount of new or exotic physics, and there are some strong theoretical arguments that support them, too. But we still need to resolve this issue experimentally. No matter what the explanation of dark matter is, we will learn something truly new and exciting by resolving this mystery.”
Dr. Henning also tells me that dark matter isn’t all that’s bugging physicists. Until about ten years ago, we believed that the pull of gravity would cause the expansion of the universe to gradually slow down. But when we discovered that the universe is actually expanding faster and faster, we realized something must be counteracting gravity — something must be pushing everything outward. Physicists called that something dark energy.
If dark matter has left us for the moment in the dark, at least we have some ideas about where to look for the light switch. With dark energy, we’re not yet sure there is a light switch. Is dark energy simply the cost of having space? That is, does a given volume of space have some intrinsic, fundamental energy? Is dark energy some sort of stuff? Did we get gravity wrong? Is there some other fundamental property of space-time that we just don’t understand?
There are even scientists who theorize that the universe could be made of information: the yes-no, on-off “bit” of computer science may actually be, in a sense, the fundamental particle of the universe, which may itself be one gigantic computer. This sounds weird until you understand quantum physics — which I don’t.
Anyway, back to the big picture: current thinking is that stars and the like account for only about one half of one percent of our universe. Another three and a half percent is intergalactic gas. About twenty-three percent is dark matter, and the rest — about seventy-three percent — is dark energy.
Hundreds of years ago, mapmakers wrote “terra incognita” — unknown land — on any place they didn’t know about yet. Ninety-five percent of our universe is terra incognita. Next time you meet a cosmologist, buy him a drink.
^ back to list
2. We’ve got the genes… now what?
Kelly Hogan in Biology sent me this question.
In 2003 researchers finished sequencing the human genome. They created a map of our entire genetic material. Much hand shaking, back patting, and confetti ensued. Okay, probably not all that much confetti. But sequencing our genome was a landmark moment. Science magazine called it a “trip-to-the-moon logistical effort.”
Now we’re starting to realize that maybe the party was premature. Sure, we have a map, but it’s like having one that shows only the general outline and topography of the United States, when what we want is a road map to Aunt Mildred’s house. As Kelly told me, we have the genome sequenced, but we don’t know what much of it means for us.
Remember what we talked about earlier: genes are more complicated than we thought. They interact with each other, and with other cellular workhorses such as RNA. But we still don’t know how genes and all these other players interact, even when it comes to some of our most common diseases. If there’s a silver lining in this, it’s that understanding genes may turn out to be a lot easier than understanding the proteins that our genes crank out.
Proteins, see, do most of biology’s heavy lifting. Before a protein can do any cellular work, it has to assemble, or “fold,” itself into one of an almost infinite number of shapes. Let’s take a very short and very simple protein made of a one-hundred-amino-acid chain. Now let’s say there are three different ways each link in the chain can fold. To find the total number of possible configurations this protein could end up in, we’d have to multiply three times three one hundred times. We’d get a number that’s roughly the current age of our universe, squared. But somehow our little protein already knows what shape to fold into, and how to fold, and then it does fold — in microseconds.
But before cells can produce proteins, they have to transcribe DNA into something called pre-messenger RNA. Pre-messenger RNA molecules are then spliced — little sections are cut out and then recombined in different ways — to create mature messenger RNA. This mature stuff then gets translated into a protein. One RNA can be spliced several different ways to yield different proteins, and different variants of the same protein are often found in different tissues, where their subtly different sequences allow them to do specific jobs. In fact, it’s impossible for us to count how many different proteins there are in humans. And if all that isn’t complicated enough, sometimes the little buggers pair up and work in teams.
Remember the non-coding DNA we talked about earlier, the stuff that scientists once thought was junk? Did I mention that it makes up about 99 percent of our DNA? We’re learning how to read it, but we’ve just started.
So you can see how we’re a long way from having a truly useful genetic map. I’m going to put Kelly on the spot here and ask her to stand up and tell me what a map like that would let us do. Kelly?
“Okay. Let’s see: some day you may be able get a readout of the exact variants you have for different disease-causing genes. And we may someday interpret that readout as a risk value for susceptibility to complex diseases that involve multiple genes. But don’t forget that your environment will always play a role. So will we also be able to pinpoint environmental conditions that add or take away risk?”
Thanks, Kelly. Let me see if I can sum this up. If we can come up with a set of rules to explain how our genes can create different proteins, in different amounts, in different circumstances, then our map may be a little more complete. But it’s going to be a while before we can use it to get anywhere.
Now for the question that you all voted the most interesting and most pressing of all. Everybody ready? Everybody still awake? Here’s our number-one question:
^ back to list
1. What will replace petroleum?
Jose Rial sent this to me. Cheap oil has spoiled us. In not much more than a hundred years, we’ve burned through a quantity of oil that took millions of years to accumulate. Almost all of our transportation here in the United States depends on oil. It’s looking like we’re going to have to make a choice: either come up with a different liquid fuel, or start figuring out how to power all our vehicles with electricity.
Some people think that biofuels — made from corn, sugar cane, and other plants — may help wean us from petroleum. But as Dr. Rial told me, relying too heavily on biofuels would create a new dilemma: do we want to move, or do we want to eat? If we’re going to stick with liquid fuel, Dr. Rial says he might put his money on gas hydrates — methane gas molecules that are essentially trapped in ice under ocean-floor sediments. There’s a lot of gas hydrate on Earth — up to ten times the amount of known reserves of natural gas. But it’s difficult to extract, and methane and global warming go together like matches and dynamite.
Right now, the world is using about 82 million barrels of oil a day, and that number seems to keep going up. Experts project that we’ll use 100 million barrels a day by 2020, and CEOs of some of the biggest oil companies have already announced that they’re going to have trouble meeting that demand. How long till the last drop? It depends on who you ask, and on how fast developing countries continue to grow. It’s not unreasonable to think we’ll be out of oil in forty-five years. Pessimists say thirty.
There are immense reserves of a few unconventional sources of oil: tar sands, heavy oil, and oil shales, for example. But it takes a lot of energy and money to extract and refine them; it’s difficult to ramp up their production to high volumes; and their greenhouse costs are high: up to three times more emissions per barrel than refining conventional oil.
If we started powering our cars, planes, ships, and trains with electricity, then nuclear energy, solar and wind power, and battery power may become good bets. But imagine the work it would take to make all our transportation run on electricity.
So, Dr. Rial, this sounds pretty bleak. Any good news for us?
“Well, this is a huge, fundamental problem, and only a few decision-makers are paying it the attention it deserves.
“At the same time, if we’re really optimistic, maybe the best way to look at this is to say that the Stone Age didn’t end because we ran out of stones. That is, some new technology will come along that will render our carbon-age technology obsolete. The internal combustion engine is the machine from hell. We’re smarter than that — I hope.
“Right now, we don’t know the answer. But wasn’t that what you wanted anyway?”
Fair enough. Thanks, Dr. Rial.
And thanks to all of you who thought about these problems, and sent me questions, and put up with my questions — and after all that, even showed up today. I’ll wrap up with this: back around 1880, a lot of scientists felt the big questions were pretty much answered. Some physicists thought all that was left was to sharpen their ideas to slightly finer points — to measure certain constants “to another place of decimals,” as they put it. Seems quaint, doesn’t it? And in a 1997 book called The End of Science, John Horgan interviewed a lot of big-time scientists, who lamented that the best and most exciting discoveries had already been made — that all the big questions were behind us.
Now I don’t know about you, but I’d love to come back a hundred years from now to see what we don’t know then. Good afternoon. And good luck.
^ back to list