“Mucus,” says Richard Boucher, “is a bit like flypaper.” Its stickiness traps the particles that are present in the air we breathe. But at UNC-Chapel Hill, mucus has also attracted mathematicians, computer scientists, and polymer chemists.
It started about three years ago when Boucher and his colleague Bill Davis wanted to understand more about the stickiness of mucus. In 1998, they published in the journal Cell that the normal lung surface was composed of two fluid layers: the mucus and a watery lubricating layer; this had major implications for cystic fibrosis (see “a new angle on CF”). “But,” Boucher says, “we didn’t know why these layers formed and why they didn’t mix.”
To help them understand, Boucher’s team looked for help. They contacted Richard Superfine, professor of physics and astronomy, who has expertise in very fine imaging. Particularly, they wanted to know about an atomic force microscope (AFM) they knew Superfine had.
Essentially, the AFM allows images to be taken at the molecular level, but only on the surface. The AFM allowed Boucher to see what the molecules that make up the mucus — the mucins — look like. Boucher says, “The mucins had some attachments that looked like bottle brushes and others that were naked. The naked attachments were very hydrophobic — water repellent. We think these are what is sticking to the cell surface.”
So the team had gained some understanding of the interactions of mucus on the lung surface. But, Boucher says, “we needed to understand the forces of the interactions.” They realized they must mimic or model this situation theoretically. That required an applied mathematician.
Boucher and Superfine went to Greg Forest, associate chair of applied mathematics and an expert in complex fluids. Forest says, “I had never considered mucus before, certainly not scientifically.” But as luck would have it, Forest’s group included people with the broad expertise required to model and simulate the transport of biological fluids. In some ways, Forest says, “our group was made to study mucus.”
While they were tackling these problems, it occurred to Superfine that “there was something bigger that we could be doing here.” He thought about it for a couple of months and then, in November 2003, sent out an e-mail to a group of chemists, mathematicians, polymer physicists, and computer scientists. He outlined the problem and the expertise they all had, and he set a much bigger goal: a fully interactive, predictive computer simulation of the human lung.
His reasoning in setting such an ambitious target was simple. “If you went into a facility where they design aircraft, you’d be amazed at the amount of mathematical modeling done before it ever leaves the ground,” Superfine says. “Why don’t we demand that same simulation firepower behind our medicine design and development?” That is exactly what the group is doing.
This approach would have an enormous impact on drug design and discovery. Currently, it takes an average of twelve years and costs nearly $900 million to bring one drug from the laboratory to the patient, according to a study in the March 2003 issue of the Journal of Health Economics. The team’s virtual lung could test thousands of drugs and their interactions before trying them out on humans, dramatically speeding up the process and lowering the costs.
But how do you go about making a computer model of a lung? To model something you first have to understand how it works. “You have to divide and conquer,” Forest says. “You want to know the basic physics of what is going on, what are the constituents, who the players are.”
“The challenge of the lung is to bring the blood into intimate contact with the oxygen you breathe in,” Superfine says. Doing that requires a large surface area, so the lung has lots of branches, like an inverted tree. “That increases the surface area to about the size of a tennis court,” Superfine says.
But that’s a huge interface with the environment. And it doesn’t contain only oxygen; it also includes contaminating particles, dust, pollen, and bacteria to name a few.
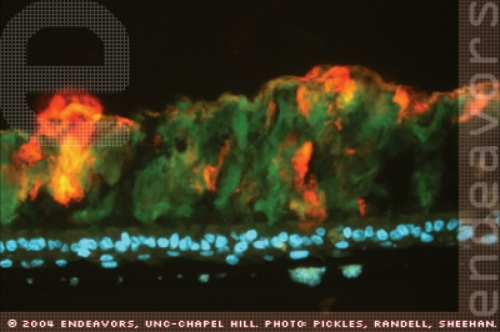
Courtesy of Ray Pickles, Scott Randell, and John Sheehan.
A lung section from a cystic fibrosis sufferer. The different mucin molecules that make up the mucus are stained red and green. The underlying cells that line the lung — epithelial cells — have their nuclei stained with a blue dye. Here, because of cystic fibrosis, the mucus is too thick to allow normal breathing and is attached to the airway surface. This adherent mucus plaque provides a rich breeding ground for inhaled bacteria to grow and to produce infection. Immunofluorescent light micrograph, magnified forty times.
Click to read photo caption. Courtesy of Ray Pickles, Scott Randell, and John Sheehan.
This is why the surface of the lung is covered in the flypaper-like mucus. Between the cells and the mucus is the very thin watery layer, only seven thousandths of a millimeter thick. This layer allows the mucus to slide across the lung surface. Boucher likens it to kids on a beach with sliders: “They get on the edge of the beach and water, and hop on their board and go forever on just a very thin film of water,” he says.
The cilia normally help clear the mucus. They act like little oars that stick out of the cells and through the watery layer and into the mucus. Each cell has about 120 cilia, which beat back and forth up to thirty times per second. This beating propels the mucus up to the throat, where it is swallowed and removed through the digestive system.
So the researchers are trying to understand how these components all work together. Forest says, “We also must have a fundamental model of the biochemistry, which is eventually going to tell us how the body is regulating itself.” Superfine likens this to understanding the thermostat in your house. “If you are cold, the heater turns on, but it doesn’t stay on. There is something measuring the temperature, and when it gets warm, the heater shuts off.”
For the computer programmers the challenge becomes how to make a computer simulation of all this. Potentially, in the virtual lung, each one of the components will have one, or several, computer programs associated with it. “Understanding how you take these separate programs and put them together and have them talk to each other is a major challenge in computer science,” Superfine says.
And for each member of the team, there are challenges. Mucus, for example, is not just a viscous liquid; it is also elastic. This means it has a dual personality as a solid and a liquid, which changes depending on how you tug on it or move it with cilia. “There are,” Forest says, “libraries of equations from the last fifty years of research in polymers, none of which have yet provided a faithful representation of mucus. The knowledge of the equations of visco-elastic liquids is still quite primitive.” So the researchers need to understand more about the components, as well as how they work together, to make headway on disease and disorders.
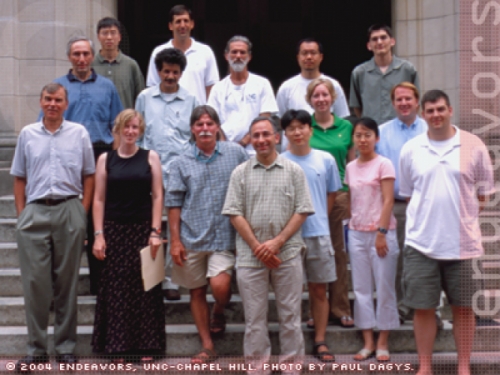
Photo by Paul Dagys.
All the members of the Virtual Lung Group.
Click to read photo caption. Photo by Paul Dagys.
The researchers do have one big advantage though — an experimental model of lung tissue in which they can test their computer models. Boucher’s group developed the model by culturing lung cells (taken at the time of lung transplant from the donor) in a Petri dish. The cells produce mucus, create the thin watery layer, and sprout cilia that beat. Then the mucus flows. “In my lab,” Superfine says, “to be able to put lung tissue under the microscope and measure forces on things has allowed us to really get at the problems.” You can also do things that you couldn’t do to a human, such as remove the mucus. Superfine says, “To my mind, having that experimental system is really what makes this whole thing fly.”
So how close to their goal are they? In ten years, if the researchers get everything they want, they envisage sitting at a computer terminal and being able to see a lung. You could enter different patient profiles — asthma, for example. Then you could enter information about a new drug. As the simulation runs, “you could zoom in at different levels and get all kinds of information,” Superfine says.
For now, the team is waiting to hear if its application for independent funding for the project has been successful. The National Institutes of Health recently launched its Roadmap initiative to accelerate medical discoveries. And the virtual lung project, because it is a large interdisciplinary group, “is the type of project that typifies what the Roadmap should do,” Boucher says.
In the meantime, the researchers are clearly enjoying working as a group. For Boucher, “it is a privilege to a bunch of us MDs to have mathematicians, chemists, physicists, and computer scientists get interested in mucus.”
For Superfine and Forest, the payoff comes in getting involved in a project that has implications for people’s health. “I give talks all the time on nanotechnology, and no one has ever thanked me personally for a talk,” Superfine says. “But I gave a talk on cystic fibrosis, and this guy comes down to me and thanks me for the work on cystic fibrosis, pulls out his wallet, and shows me a picture of his daughter. She has cystic fibrosis. Two months later I get a Christmas card from her.”