Want to see a bunch of astronomers wired like kids on Christmas morning? Want to see them on cloud nine, in seventh heaven, and — work with me here — with stars in their eyes? Tell them they can design and build a world-class telescope. Then go away and let them do it.
The astronomers in Carolina’s Department of Physics and Astronomy, with a little help from their friends at the National Optical Astronomy Observatory, Michigan State University, and the countries of Brazil and Chile, have spent the last eighteen years cooking up a high-tech telescope called SOAR (Southern Astrophysical Research). They’ve thrust the thing far into the sky in the foothills of the Chilean Andes.
It isn’t the biggest telescope on the planet, but SOAR just might prove one of the world’s most productive. It will capture the highest-quality images of any observatory in its class in the world. It will be more versatile and efficient than most telescopes. And Carolina’s astronomers will be able to point it wherever they like without ever leaving Chapel Hill. With SOAR, Carolina plans to become a key player on the global astronomical scene.
Near the Top of the Bottom of the World
Cerro Pachon is a dusty, nine-thousand-foot desert mountain about thirty miles east (as the condor flies) from the town of La Serena on Chile’s Pacific coast. Down in La Serena, all the taxis, stray dogs, and uniformed schoolkids running around make the place seem busy. But up on Pachon, it’s absolutely silent, and your neighbors come in two flavors: mountains (with Pachon’s sister foothills nestling all around and the Andes proper piling up in the distance) and telescopes (from Pachon, you can’t see anything man-made that doesn’t have to do with astronomy). SOAR’s clean white dome looks a little improbable up here, like a gigantic golf ball dropped in the desert.
Why Chile? Well, the Southern hemisphere has fewer telescopes than we have up north. And astronomers, as the bumper sticker says, do it all night. They need clear, dark skies. The Chilean coast has relatively few cloudy nights, little rain, and not much ambient light from cities and towns. Add to that the center of the Milky Way galaxy passing right overhead, and Chile becomes an astronomer’s heaven.
Looking into the Big Glass
SOAR moves like a battleship gun: the entire dome can rotate, and the four-meter mirror can move up and down. The mirror itself is about four inches thick and polished to a slightly aspherical shape (to a tolerance of about seven billionths of an inch). It’s supported by pads connected to 120 electric motors, which can independently push and pull the mirror from below so that it keeps the same shape no matter which way it’s pointed (seven thousand pounds of glass act weird when you start moving them around). The mirror’s reflectivity — and to some degree, the telescope’s effectiveness — depends on an aluminum coating only three millionths of an inch thick. In fact, you could say that the $30 million, one-hundred-ton SOAR telescope is really just a skeleton to hold a couple tenths of an ounce of aluminum — a few pennies’ worth — precisely where it needs to be. SOAR’s reflectivity at this primary mirror is around 91 percent, about the best the astronomers could have hoped for.
When it comes to images, SOAR delivers. There’s a trade-off in telescope design between the amount of viewable sky and the quality of the resulting image. Generally, the more sky you can see, the poorer the image quality. SOAR captures relatively small slices of the sky at very high image resolution. It can detect celestial objects about a billion times fainter than the naked eye can see.
SOAR is quick on the draw, too. For light to be useful to astronomers, the telescope needs to pass it through one of several instruments — such as a spectrograph or an infrared camera — which process the light in various ways. At many other telescopes, changing an instrument takes hours or even days. But SOAR’s eight different instruments are set up like a railroad switchyard, allowing astronomers to redirect light to any one of them within a couple of minutes.
The Homesteader
Wayne Christiansen’s grandparents emigrated from Denmark and settled in Fort Collins, Colorado. In exchange for working on a government irrigation project — Christiansen’s grandfather dug, and his grandmother cooked for the workers — the couple settled on a plot of land near the water lines, knowing it would be fertile. They built a cattle farm that’s still in the family today.
When I catch up with him in Chapel Hill, Christiansen is about to head out to Fort Collins for a few weeks’ work on the farm. He’ll stay in what the family calls the little red house, a one-bedroom cabin that “doesn’t have a full-fledged, female-approved bathroom,” as Christiansen puts it. (“It does have running water,” says Christiansen’s wife, Judy. “That’s the only reason I put up with it.”)
At sixty-three, Christiansen has the look of someone who might have been a high-school sports standout, and you can read the farm work in his hands and face. Among other things, Christiansen studies the movement of galaxies — how fast and in what direction they’re going. The universe is expanding — faster and faster, as astronomers recently found out — and most galaxies can generally be said to be moving away from us. Christiansen combines data from different kinds of telescopes, including radio, X-ray, and optical, to build up a clear idea of what a galaxy cluster such as Abell-3128 is up to.
In 1986, when Chancellor Christopher Fordham encouraged faculty to come up with high-profile research projects, Christiansen swung for the fences. He went immediately to Bruce Carney, Carolina’s only other astronomer at the time, and told him — chutzpah firmly in place — that they should build a telescope. Eighteen years later, Christiansen is ready to SOAR.
The ability to get data from SOAR as astronomical phenomena happen will be worth its weight in gold for Christiansen. Typically, astronomers submit competitive proposals, up to a year in advance, for telescope time. Agencies such as the National Optical Astronomy Observatory then award time on the nation’s telescopes. Even if your proposal is awarded, it might be months before you can get on a telescope. And you’d better hope for clear skies, and that nothing is happening that’s more interesting than what you originally proposed to do. “You don’t just go in and pop off a red shift,” Christiansen says, referring to a measurement that tells astronomers a galaxy is moving away from us.
But now Christiansen can pop off red shifts — and blue shifts, and almost anything else he cares to look for — on one of Carolina’s 124 half-nights per year of observing with SOAR. Like his grandparents, he has settled in just the right place, and when all that light from long ago comes trickling down into SOAR, he’ll be ready.
The Romantic
Astronomers will tell you that stars don’t really twinkle. (It’s just an effect caused by the earth’s atmosphere.) So something else must be putting the sparkle in Bruce Carney’s eyes. He’s a bit of a romantic: he likes to point out that one of the agreements committing the university to SOAR was signed atop a pier that Joseph Caldwell built in 1824 to hold the university’s first telescope. Carney wore the same necktie (printed with Van Gogh’s Starry Night) to both the SOAR groundbreaking and the official SOAR dedication, which happened six years — to the day — apart. And when it comes to his research, Carney is fond of sticking close to home.
Carney studies our own galaxy, the Milky Way. Some of the Milky Way’s oldest stars are near its center. All the dust between us and those stars makes them hard to see: in visible light, Carney says, they’re three hundred thousand times dimmer than they are in infrared light.
So he attacks in infrared, where factors such as dust and moonlight don’t make much difference. “Infrared astronomers are bottom-feeders,” Carney jokes. “We’re the catfish of the night. As long as we’re getting light, we’re happy.” But by getting data on the temperature, brightness, and chemical compositions of our galaxy’s stars, Carney can start to deduce their ages. “You can get some idea about whether the Milky Way took a billion years to form, or several billion years,” he says.
The better the image quality, the more Carney can tell about the stars he studies. SOAR’s images won’t just be good, they’ll be great — on the order of what the Hubble Space Telescope can do, if over a smaller field of view. SOAR’s emphasis on image quality and aperture (the amount of light it can bring in) will make it a world leader in high-resolution astronomy images.
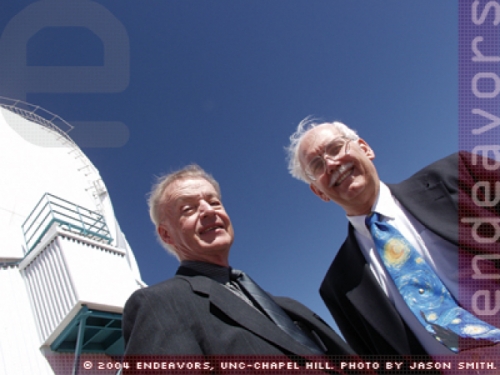
Photo by Jason Smith.
Wayne Christiansen and Bruce Carney with the SOAR telescope in La Serena, Chile.
Click to read photo caption. Photo by Jason Smith.
Carney remembers the day Christiansen had his big idea. Carney had a date that night: dinner at Crook’s Corner with Ruth, to whom he’s now married. He was excited to tell Ruth about the telescope over dinner. “She gave me neutrally positive comments about it,” Carney says carefully, “but she was sort of inwardly rolling her eyes the whole time.”
The Remote Controller
Gerald Cecil was SOAR’s project scientist. But he’d be the first to admit that the control room down in Chile isn’t going to knock your socks off. It’s not 1950s sci-fi, he explains: no futuristic knobs or buttons, no glass balls buzzing with mysterious light. It’s just a narrow room one floor below the telescope mirror, and it holds a handful of normal desktop computers and a TV monitor or two.
Then again, what you can’t see from the control room is what Cecil is most proud of: SOAR’s observatory, telescope, and instruments are controlled by computer systems more advanced than the control system of any other telescope on the planet. The programs that control SOAR are easy to understand, Cecil says, and they will adapt easily to changes that happen as SOAR’s mission evolves.
Anyway, Carolina’s astronomers won’t mind the lack of whiz-bang in the control room up on Pachon — after all, they’ve got their own (see “The Undergraduate Behind the Controls”). They can be on SOAR and not at SOAR. Cecil made that happen.
“Really, when you’re down in Chile, you rarely go upstairs to deal with your instrument,” Cecil says. You merely deal with the stream of data it gives you, he explains, so you might as well do that in Chapel Hill. And when you need to know something about the sky or weather conditions, being five thousand miles away might just be an advantage. “Down there, the temptation is to just go out and look up,” Cecil says.
But up on the big screen in Carolina’s remote observing room, astronomers will “see” the cloud cover, the positions of the Milky Way and other celestial objects, and the weather conditions much better than they could with their own eyes in Chile. Their celestial targets will be superimposed on the night sky as it inches by. As the astronomers track these targets, they’ll tell the telescope operator down in Chile how to react to the changing weather, cloud cover, and the moon.
Cecil’s been working for years on outflows from galaxies. “Most of the motions in galaxies are boringly predictable,” he says. “The stars go around the center in these one-hundred-fifty-million-year orbits, chug-chug-chug, living and dying, and some blow up, whatever.”
But some galaxies intrigue Cecil a little more. He gets out a picture of galaxy NGC 3079, which has ejected a gigantic bubble of gas — about three thousand light-years across — that might be escaping NGC 3079’s gravitational pull. That would send chemically enriched gas into contact with the primordial hydrogen of intergalactic space, which would theoretically affect the evolution of stars. But Cecil is just trying to learn why the gas flows as it does, and what causes these massive eruptions, called superbubbles.
Cecil wants to know if superbubbles occur in isolated galaxies, or if something has to alter the gravity of a galaxy — such as another galaxy passing nearby — before a superbubble can happen. Superbubbles are a crucial connection between the life cycles of stars and the evolution of galaxies, he says.
“And there are striking hints that the same sort of phenomenon is going on in our own galaxy,” he says.
The Light Splitter
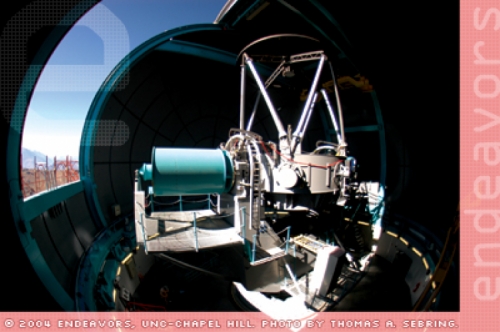
Photo by Thomas A. Sebring, SOAR project manager.
The SOAR telescope ready for action.
Click to read photo caption. Photo by Thomas A. Sebring, SOAR project manager.
Chris Clemens built SOAR’s Goodman Spectrograph, the instrument that will separate incoming light into different wavelengths. This isn’t something you can put together with a Home Depot kit and a hot glue gun: there are only around ten spectrographs in the world like SOAR’s. Clemens was hired specifically to build it. It took him the better part of two years’ thinking and four years’ work.
Without a spectrograph, a telescope is just taking pictures, which are useful to an extent. But a telescope’s image of a star is really just a brighter version of what you can see with your eye. To really know something about a star — how it’s spinning, what it’s made of, what its surface gravity is like, and whether it has a magnetic field — you need to split up the light. For much of the history of astronomy, that’s been a wildly inefficient process. Before the 1970s, spectrographs were operating at only 3 percent efficiency. “You went through all that trouble and expense to collect the light, and you threw away ninety-seven percent of it,” Clemens says. Efficiency jumped up to 70 or 80 percent in the 1970s, but the dispersers — the part that actually splits the light — didn’t improve at all. Now, by making something called a volume holographic grating, Clemens has just pushed his spectrograph’s visible-light efficiency up to around 60 percent.
SOAR’s spectrograph also holds another trump card: at around 50 percent, it’s currently the most ultraviolet-efficient spectrograph in the world (the competition is as low as 3 percent efficient in the UV). Ultraviolet spectroscopy is a narrow research window, but one that holds interesting things for many astronomers.
Clemens will use SOAR to study stellar seismology. Certain stars, called variable stars, regularly change in brightness. This may be due to seismic disruption: basically, starquakes. By using time-resolved spectroscopy, which involves getting a lot of spectral “snapshots” over a certain period of time, Clemens can learn something about what’s inside these variable stars.
But astronomers like Clemens really want to know about fundamental stellar physics: what’s holding stars together? In some stars, it’s air pressure or gas pressure, just like in a balloon. But the physics get trickier in denser stars. “We can’t study any of that stuff in a lab; we can only study it in space,” Clemens says.
Even so, what goes on way out there and what happens on the lab bench are more closely related than you might think. Physicists first began to study nuclear reactions because astronomers knew the reactions must be going on in stars. “There’s a definite connection between what we do and what physicists do in the lab,” Clemens says. “Our lab is just bigger.”
A Universal Architect
James Rose wants to know how our universe got the structure it now has. Most astronomers believe the universe was originally gas — mostly hydrogen and helium — and that it somehow became filled with galaxies, galaxy clusters, and even clusters of clusters of galaxies (called superclusters). There’s a hierarchy here: smaller objects such as galaxies tend to form first. Those may later interact to form larger objects, such as clusters. Or as Rose puts it, “it’s all smaller lumps forming bigger lumps.”
Individual galaxies move around within galaxy clusters like bees inside a swarm. These clusters are often saturated by one-hundred-million-degree goop — a gas, actually — called intergalactic medium. When a galaxy plows into this intergalactic medium at two and a half million miles an hour, the heat and resulting shock wave can push the galaxy’s own gas right out. Any gas that doesn’t get pushed out can get compressed to form new stars. So when astronomers see a lot of star formation along one edge of a galaxy, they suspect intergalactic medium might be wreaking its havoc.
Rose studies Pegasus I, a cluster of galaxies 150 million light-years from Earth (right in our back yard, as clusters go). Pegasus I isn’t behaving. “It’s kind of a ratty little cluster,” Rose says. “The galaxies are not moving around very fast, and there’s not much intergalactic medium there.” Yet some galaxies in Pegasus I act like they’re moving through intergalactic medium — they appear to be losing gas and forming new stars along one edge.
Rose would like to find out why. He uses all sorts of data sources, including X-ray observations, but it really helps to have good images and good data from the spectrograph. SOAR should be able to give him both, and he won’t have to wait for telescope time to get them.
The Whiz Kid
If you were an undergraduate and Dan Reichart moved into your dorm, you wouldn’t think anything of it. He wouldn’t even need to put up the Star Wars poster that hangs in his office. He might pass for a student — Christiansen and Carney have been working on SOAR since Reichart was twelve — but Dan Reichart is actually a rising star among astronomers. And he studies a sexy topic, as astronomy goes.
Gamma ray bursts are cosmic explosions. They’re so bright that we can detect them from far away — in fact, astronomers figure gamma ray bursts are the most distant detectable things in the universe — and they illuminate whatever’s between us and them. Reichart wants to use gamma ray bursts to backlight the universe and get new clues about how it formed.
But until recently, gamma ray bursts were leaving us in the dark. We didn’t know where they came from or what they were. In 1997, scientists discovered that gamma ray bursts were coming from deep space. Then in 1999, Reichart was one of three scientists to discover that these cosmic explosions happen when stars at least thirty times larger than our sun die off.
There’s only one catch: to see a gamma ray burst, you have to be quick. They peak within minutes, sometimes seconds. With existing technology, Reichart might not be alerted to a gamma ray burst for hours, and by then, most of the interesting data are already gone. But Reichart is building six small telescopes to chase these intergalactic ghosts. If his scopes pick up a burst, and it turns out to be something interesting, Reichart will be able to interrupt SOAR.
Here’s where SOAR’s versatility can really pay off. If a gamma ray burst happens while SOAR is getting optical data, the telescope operator can redirect the light to SOAR’s infrared instruments — which are used to see gamma ray bursts — in under a minute. SOAR moves pretty quickly, too. “Within two minutes I can get anywhere in the sky,” Reichart says. “Let’s say we find the most distant thing in the universe. If Keck — the largest telescope in the world — doesn’t have the infrared on, they’re out of luck.”
The Space-Time Trailblazer
If you can’t find Charles Evans, look in the woods. He just might be off leading a Boy Scout camping trip with one of his sons. And though he might not be the first to bring it up, Evans has quietly blazed his own trails in theoretical astrophysics, Bruce Carney says.
Evans likes to think in relative terms: when two black holes encounter each other, they distort space-time and send ripples of gravity through the universe. At that point, Isaac Newton’s gravity isn’t a good enough compass to get astronomers out of the woods. So they turn to Einstein’s general relativity. “The equations are nasty to the point of almost always being intractable, incapable of solution in any traditional sense,” Carney says. But Evans has helped pioneer ways of calculating numerical solutions.
Gravitational waves — those space-time ripples caused by black holes and other objects — are hard to detect from earth. So hard that the Laser Interferometer Gravitational Wave Observatory (LIGO) built two detectors, each with a pair of “arms” that are four kilometers long. Any gravitational waves headed our direction minutely change the lengths of those arms. LIGO uses lasers to measure these giant arms as their lengths change — to a precision of less than one one-thousandth of an atomic nucleus. But even when LIGO does detect gravity waves, we have trouble telling what caused them. Evans’ theoretical work is meant to help sort that out.
When it’s time to SOAR, Evans’ knowledge of black holes will dovetail nicely with Dan Reichart’s study of gamma ray bursts (they’re occasionally associated with black holes). Since these bursts fade so fast, the two will work together to learn as much as SOAR can tell them about a given burst.
Evans hopes some of those bursts will be from collisions involving two neutron stars, or a neutron star and a black hole. A neutron star might have a mass one and a half times that of our sun, but squeezed into a space only ten or so kilometers across. A black hole is even denser than that. Both are remnants of dead stars. There are places in the universe where these “stellar corpses” are doing weird things. Say a black hole and a neutron star are orbiting each other. Over millions of years, they spiral closer and closer together because of the gravity waves they give off. Once their orbit shrinks to one hundred kilometers or so, these massive objects whiz by each other hundreds of times per second.
“It only takes a few minutes for the end to come once they get that close,” Evans says, “and it’s a spectacularly violent event.”
These collisions give off enough energy that we could expect to detect them via satellite, with LIGO, and with optical observation from SOAR — getting three kinds of data on one event. “That would be the Rosetta Stone,” Evans says. “That’s when you really learn something.”
An Observer, In Theory
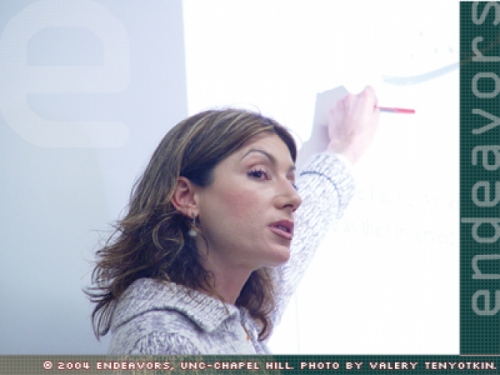
Photo by Valery Tenyotkin.
Click to read photo caption. Photo by Valery Tenyotkin.
Laura Mersini is a theoretical physicist, but SOAR helped draw her to Carolina. She says that when it comes to what we know about the universe, current physics theory is lagging a little behind. For example, the Big Bang theory might not be enough to explain the origin of all the matter in the universe (everything from the universe’s large-scale structure — galaxies and the like — to cosmic microwave background radiation, dark matter, and the rest). But theory can’t catch up without observation. “We need new physics, and that’s exactly why we need SOAR,” she says.
The Governor’s Man
When Robert McMahan is wearing his astronomer’s hat, he talks about what SOAR can do for science and what he might like to do with SOAR. When he’s wearing his Raleigh hat as N.C. Governor Mike Easley’s senior advisor for science and technology, he talks about SOAR as a long-range, strategic investment for the university and the state. Building new instruments for the telescope means pushing new technologies, he says. “And SOAR can become a catalyst for the university to do much more,” he adds — to inspire students and expose them to cutting-edge technology, to create a scientific infrastructure that brings the brightest people and best ideas together, and to increase Carolina’s visibility internationally.
Take Chris Clemens and his volume holographic grating. They’re now in high demand for other telescope projects. Clemens has designed gratings for the world’s largest telescope, called TMT (Thirty-Meter Telescope). “Our technology is at the core of every project from now on,” Clemens says. “We’ve more or less conquered a technology that people need. We’re building not only science, but a technological expertise. And that expertise is going to take us wherever we want to go.”
Back under his astronomer’s hat, McMahan has spent a good bit of time studying the large-scale structure of the universe. Galaxies, as it turns out, aren’t evenly spread through our universe, but instead occur in the “interstitial regions of large voids,” McMahan says. Think of soap bubbles in your kitchen sink. Galaxies tend to be distributed through the universe as if they were lying on the surfaces of those bubbles, without much stuff in between the galaxies (inside the bubbles).
But SOAR’s got McMahan thinking again about something he worked on early in his career: white dwarfs. They’re the small cores of dying, low-mass stars that cool off slowly, over billions of years. Once they do cool, they’re very faint: when the Hubble Space Telescope found seventy-five white dwarfs in the Scorpius constellation, the brightest of them was about as dim as a one-hundred-watt light bulb on the moon as seen from Earth. “The hotter stars are well-observed,” McMahan says. But white dwarfs are a little neglected. SOAR can help McMahan begin to change that.
SOAR has been near and dear to McMahan since he got to Carolina in 1989. Did McMahan ever think SOAR wasn’t going to happen? “Oh, gosh, lots of times,” he says. “Sometimes it was just sheer pig-headedness that got the project through, and a desire not to let it go.”
Pig-headedness or not, it has paid off. Carney feels SOAR is a quantum leap for Carolina. He and Christiansen can remember when supplies were going up to Cerro Pachon by horse and mule, and how the bulldozer driver they hired to cut the road to SOAR had worn out one of his blades only halfway up the mountain. Fast-forward fifteen years to April 2004, just before SOAR’s dedication ceremony, when Carolina’s astronomers held a V.I.P. videoconference from SOAR’s control room in Chile back to Chapel Hill. “Wayne Christiansen had a smile on his face a mile wide,” Dan Reichart remembers. “You could have mugged him and not wiped that smile off his face.”