On the northern tip of Ireland, where the North Sea meets the Atlantic, waves smash into 40,000 ancient, hexagon-shaped pillars at Giant’s Causeway — the result of a volcanic eruption 60 million years ago. Small pools form in the cracks between each column. As the winds rage, the sun scorches the landscape, and a bone-chilling sweat settles over Laura Mersini-Houghton, who shifts uncomfortably. Across from her, a documentary filmmaker stands behind a video camera and asks her a question. She tries not to shiver as she replies.
“Think of these waves leaping over the rocks as the wave function of the universe trying to travel through this landscape structure. If I think of the rocks as the energy field and each pocket representing an energy valley on the landscape, as the waves come through, many will be trapped in different pockets rather than travel further. Each pocket can be a birthplace for a universe similar to ours.”
Mersini-Houghton is explaining the multiverse hypothesis. First suggested by the Ancient Greek philosopher Democritus, it is the idea that our universe is one of many formed by a Big Bang-like event — a concept that Mersini-Houghton agrees with. A UNC cosmologist and pioneering theoretical physicist, she began studying the origin of the universe decades ago. Why? Because the probability that our universe should even exist is basically zero, according to Mersini-Houghton. “The chances are one in 10 with 123 zeroes behind it, so pretty much zero.”
In 2005, Mersini-Houghton had a curious idea. A frequenter of coffee shops, she sat in quiet contemplation at the Starbucks on Franklin Street, staring thoughtfully at her pen and pad on the table. She began to think about the mathematical representation of the universe, which forms a wave, and spent hours lost in thought over it.
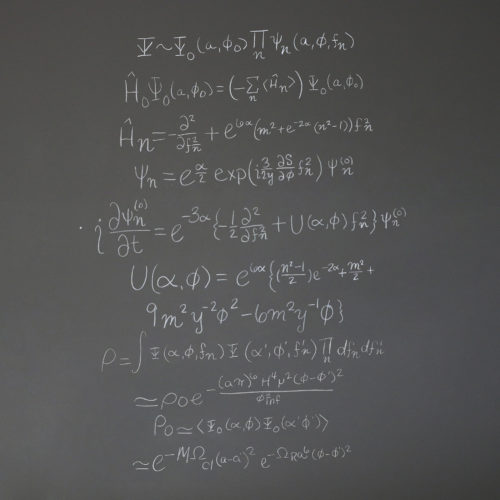
From a mathematical perspective, this is Mersini-Houghton’s calculation for the origin of the universe.
Then something clicked: What if she combined the physics of string theory (the idea that matter and energy are composed of tiny, vibrating strings) with those of quantum mechanics (how matter and light behave at microscopic levels)? She used the former to manipulate the wave, and after calculating how that waveform evolves, she found that the result is many universes — each with their own properties and constants — and one high-energy Big Bang. To the average mind, the process sounds complex, but to Mersini-Houghton, “it’s so simple that it’s too simple,” she chuckles.
After taking more time to work out the problem, Mersini-Houghton developed her first calculation for the origin of the universe. “We got the correct answer theoretically for the first time in science,” she says. “Before then, we hadn’t been able to derive an answer to the origin of the universe. That gave me hope to check for predictions to test that theory. But getting the right answer does not guarantee what nature does. What does nature care about some theory I cooked up?”
Although Mersini-Houghton is not the first person to support a multiverse hypothesis, she is the first to successfully derive the answer from basic physics principles and live in a time when there’s physical observations that support it.
The same sky
If other universes exist, then those closest to our own would exert a gravitational tug, causing matter to shift. Think of it like a piece of fabric. Pull on it from both sides and it begins to stretch. Continue tugging on it and, over time, a hole forms. For Mersini-Houghton, this meant that, somewhere in our universe, there should be a void. A big one.
“The sky we observe today is just a blown up version of the sky from 14 billion years ago,” she explains. “So whatever happened in the universe’s infancy — it’s still there somewhere in our sky. And we can track it down.”
In August 2007, University of Minnesota physicist Lawrence Rudnick did just that — accidentally. While studying data from the NRAO VLA Sky Survey, he discovered an abysmal hole, nearly a billion light-years across, containing virtually no matter. And it was located in the region Mersini-Houghton and her collaborators first predicted it would be in 2006.
“We got lucky,” she admits. “It was first observed accidentally only seven months after we predicted it. It caused huge fights in the astrophysics community about whether those observations were correct or not.”
Data from the Wilkinson Microwave Anisotropy Probe (WMAP) satellite helped verify the void’s presence. The satellite measures temperature fluctuations throughout the universe, and the hole’s location runs cold. But it wasn’t until 2015, after using a more precise satellite called Planck, when the cold spot’s existence was officially recognized and confirmed.
“I was in the right place at the right time,” Mersini-Houghton says. “When I was working on these predictions I thought we would not have these observations in my lifetime. They’re challenging our understanding of the universe — and we cannot brush them under the rug.”
What Mersini-Houghton calls luck has drastically propelled her career forward. She’s since been tenured and is regularly sought after as an expert in her field, with appearances in a variety of documentaries including the BBC’s “What Happened Before the Big Bang” (2010) and “Which Universe Are We In?” (2015), as well as the Science Channel’s “Through the Wormhole with Morgan Freeman” (2011).
Into the void
After spending 10 years tweaking her multiverse hypothesis, Mersini-Houghton shifted her attention to a popular topic of debate in the physics world: black holes. “Even today, nobody understands what happens inside a collapsing star that is about to become a black hole — especially in quantum mechanical terms,” she says.
Problems often arise here due to conflicting theories. Einstein’s theory of gravity suggests that the gravitational attraction of a black hole is so powerful that not even light can escape its grasp. But a fundamental law of quantum theory states that no information from the universe can ever disappear. And in 1974, Stephen Hawking first argued that black holes do emit a type of radiation that reduces the mass and energy of the hole. Today this is called Hawking radiation.
“But in order to have a consistent study, we need to incorporate both theories simultaneously,” Mersini-Houghton says. Physicists who tried to combine the two theories in the past were left with a mathematical mess called the information loss paradox.
In 2014, Mersini-Houghton developed a novel numerical solution to the problem, suggesting that as a star sheds radiation it also sheds mass — a process that stops the formation of singularities in the center of black holes. Singularities are one-dimensional points containing a huge mass in an infinitely small space, and they continue to vex scientists because the laws of physics cease to operate where they exist.
“They are thought as holes that pinch the fabric of space time in our universe and give rise to many speculations,” Mersini-Houghton says. “But we don’t yet know if singularities can be physically found at the center of a black hole.”
Does that mean black holes don’t exist? No, it means that quantum effects can prevent the formation of a singularity at the center of the black hole.
“It’s a difficult problem,” Mersini-Houghton stresses. “And I wasn’t completely happy with my model, which made a lot of approximations. So I decided to call the founding fathers in Stockholm and hold a conference.”
Collective wisdom
In 2015, in a small conference room at the KTH Royal Institute of Technology, a university in Stockholm, Sweden, a group of scientists gathered around four rows of tables, engaged in animated discussion. Some sat patiently. Some paced around the room. Others threw their hands up in frustration.
“What, to us, is passionate debating, to an outsider might look like vicious fighting,” Mersini-Houghton once told a reporter from the News & Observer.
This is how the world’s most renowned theoretical physicists work to uncover the unknowns of the universe. In August 2015, Mersini-Houghton brought 31 of them together — with help from UNC Chancellor Carol Folt, then-Provost Ron Strauss, and College of Arts & Sciences Dean Kevin Guskiewicz — to discuss black holes.
The 2015 Hawking Radiation Conference was more like “a huge brainstorming session than a conference,” she says. For six days, the greatest minds in physics — including Nobel Prize winner Gerard T’Hooft and the late Stephen Hawking — participated in dozens of presentations and hours of in-depth discussion. After all was said and done, the problem that’s stumped scientists for more than 40 years continues to do exactly that. But it did open the door to new ways to study the topic and help pass that information onto the next generation of physicists.
On the fringes
The most challenging part of this type of research is time, Mersini-Houghton says. “It’s racing against time. When I’m thinking about a problem, I can’t get it out of my head. And I love spending four, five, six, seven hours just thinking and grinding at it. Quite often, I can’t do that — I have to teach, show up to meetings, apply for grants, be a mom. I have to wait to start my science until the evening, when everything else is taken care of, and then work until 2 or 3 in the morning.”
Time has become even more limited for Mersini-Houghton since she began writing a popular science book on her work two years ago. But with such little time, why write a book? “We as scientists have not done a great job at sharing that science and enthusiasm and results with the public,” she admits. “An element of our job is to be ambassadors of science. And that’s a big motivation for the book.” But the true impetus for the book, she says, is the public’s request for one.
The book, like her own research, starts at the beginning, providing a history of ancient civilizations and their big questions about nature. Where do we come from? Why do we live in this universe? How deep does it go? “All of those questions were first answered with mythology,” she says. “You have gods and goddesses, monsters and dragons, colliding universes and worlds. It’s all driven by that same curiosity. And over time it evolved from myths to philosophy to science and technology.”
With the book slated for release next year, Mersini-Houghton has refocused her attention from the origins of the universe to its end. Specifically, she’s interested in dark energy, an opposing force that counteracts gravity and causes the universe to expand at an accelerated rate. “Nobody knows what it really is,” she says. “We know it makes up 75 percent of the universe right now, but that’s about it.”
What theoretical physicists do know is that it’s the same kind of energy that caused the Big Bang. And because it grows over time, it’s likely to be the only thing left in the universe at some point. “We’ve never come across anything like it before. It’s probably the toughest problem in physics.”
When you ask Mersini-Houghton what’s the point of all this, she’s quick to reply: “Because this is our home.”
Why did Homo sapiens feel the need to produce those beautiful drawings in the caves of France and Spain 17,000 years ago? Why did ancient cultures invest such effort in explaining, through myths, how our universe came to exist? The reason is simple: The survival of the human race depends on understanding our environment and using that knowledge to our advantage.
“There is a general awe for how our universe came to be,” she continues. “And it’s something that I’ve talked about, randomly, with people who attend my public lectures, at parties, or someone I sat next to on an airplane. It’s a question that has fascinated humans since the cavemen and is crucial to our existence. We are here now. We observe the world around us. We would like to know where it came from.”